Viral Detection and Clearance Within the Biopharmaceutical Industry
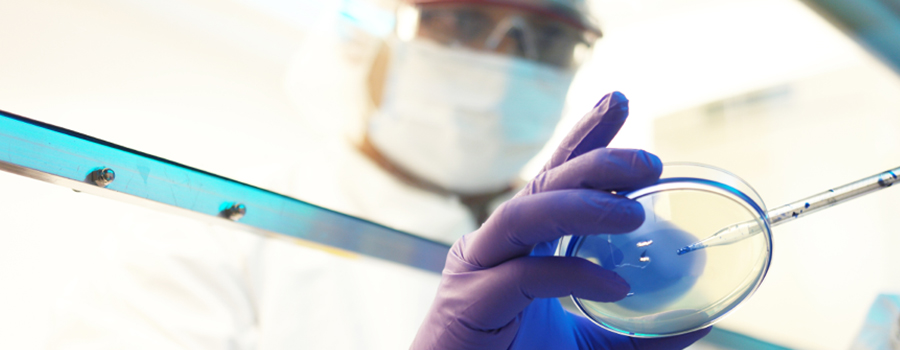
Biopharmaceutical production often includes the risk of viral contamination, which can severely impact quality, efficacy and safety. Viral detection and clearance are techniques manufacturers use to identify and eliminate viruses in biopharmaceutical processes, helping ensure that final products are free from harmful viruses.
Biopharmaceutical manufacturers use several viral detection methods to identify viral contamination, including testing cell lines, raw materials and the final product. If a virus is detected, manufacturers use viral clearance techniques to remove or inactivate it during production. The two processes, used together, help minimize the risk of contaminating products and transmitting harmful viruses to people who use them.
Here are some of the reasons viral detection and clearance is essential to biopharma production:
- Product safety. If viruses contaminate biopharmaceutical products during production, they could harm patients who use those medications. Ensuring final products are free from viral contamination is essential to maintaining safety.
- Regulatory compliance. Regulatory agencies, such as the U.S. Food & Drug Administration (FDA), have stringent requirements for biopharmaceutical production to ensure product safety and efficacy. Viral safety (ICH Q5A) is part of the regulations that companies must meet to bring their products to market.
- Quality assurance. Detecting and eliminating viruses helps maintain the consistency and quality of products batch after batch, allowing manufacturers to consistently produce reliable and effective medications.
- Protecting manufacturing processes. Viral contamination can disrupt manufacturing processes, leading to costly production delays. Companies can help prevent interruptions by implementing robust viral detection and clearance methods.
- Maintaining public trust. Thorough viral detection and clearance help ensure the safety and purity of biopharmaceutical products, which is essential for patients’ confidence that their medications are free from harmful contaminants, including viruses. Finding and eliminating adventitious viruses, which are unintentionally added during manufacturing, and endogenous viruses, which are part of the cell lines used for production, is critical for product safety. Advanced molecular techniques can help ensure any potential viral contaminants are detected and removed effectively.
Overall, viral detection and clearance techniques in biopharmaceutical production are essential for safeguarding public health, ensuring regulatory compliance, maintaining product quality, protecting manufacturing processes and upholding public trust in the pharmaceutical industry.
Viral detection techniques
Various viral detection methods are commonly used in biopharmaceutical production. Each technique has different capabilities, so choosing the best method depends on the product and process requirements.
Among the factors that should be considered when choosing detection methods are:
- The types of viruses. Different techniques may be needed to detect specific types of viruses.
- Product characteristics, including the type of cells used for production, the purification process and final formulations.
- The product’s susceptibility to viral contamination.
- Regulatory compliance and safety standards for the specific product.
- Manufacturing complexity, including the number of production steps that can each provide an opportunity for viral contamination.
- New viruses could emerge.
The detection method must be sensitive to identify low levels of viruses while also avoiding false positives.
Viral detection methods detect viruses by measuring contamination in cells or viral proteins, particles or markers. Some of the most common methods in use today include PCR, ELISA and mass spectrometry.
Learn more about viral detection techniques in this article.
Polymerase chain reaction
Polymerase chain reaction (PCR) is a widely employed molecular biology technique in virus detection. It amplifies specific segments of DNA or RNA, allowing for the high-accuracy identification of viruses. PCR is a fundamental tool that helps identify viral genetic material within samples collected from various sources.
Here’s how it works:
The first step in using PCR for viral detection is collecting samples from the product, the cells used to make it or the production environment. After sample collection, nucleic acid extraction is performed to isolate the viral genetic material, typically DNA or RNA, from the rest of the sample components. Any RNA in the sample is converted to DNA through reverse transcription, where an enzyme called reverse transcriptase synthesizes a DNA strand complementary to the RNA template.
The sample’s DNA is mixed into a unique solution containing DNA polymerase, an enzyme that makes copies of DNA. The mixture also includes primers, or short strands of DNA that stick to specific parts of the virus’ genetic material, and nucleotides that the DNA polymerase uses to create new DNA strands. Finally, buffers and other ingredients are added to make the conditions needed for the reaction. This mixture is then subjected to a series of thermal cycling stages within a PCR machine.
Thermal cycling involves three primary steps: denaturation, annealing and extension. Denaturation involves heating the sample to separate double-stranded DNA into single strands. During annealing, the temperature is lowered to allow the primers to bind to their complementary sequences on the viral DNA. During extension, the DNA polymerase enzyme synthesizes new DNA strands complementary to the viral DNA template. Through multiple cycles of denaturation, annealing and extension, the target DNA sequence undergoes exponential amplification. That results in a significant increase in the copies of the viral genetic material present in the sample.
Plaque assay
In biomanufacturing processes, plaque assays play a crucial role in quantifying viral contaminants that may compromise the quality and safety of biopharmaceutical products. These assays are essential to ensure the efficacy of viral clearance steps and validate the absence of viral contaminants in biopharmaceutical products, such as vaccines, recombinant proteins and gene therapies. By accurately quantifying viral particles, plaque assays allow biomanufacturers to adhere to stringent regulatory requirements and maintain product safety and efficacy.
During biomanufacturing, plaque assays are typically employed at various stages to monitor and quantify viral contaminants. For instance, they may assess the viral load in raw materials, such as cell culture supernatants or biological fluids used in upstream processing. Additionally, plaque assays are instrumental in evaluating the effectiveness of viral clearance strategies, such as viral filtration, chromatography and inactivation steps, implemented during downstream processing. By quantifying the reduction in viral titers achieved by these purification steps, biomanufacturers can ensure the safety and purity of the final product.
Plaque assays are valuable tools for process validation and quality control in biomanufacturing. By routinely performing plaque assays on process intermediates and final products, manufacturers can confirm the absence of viral contaminants and validate the robustness of their manufacturing processes. This proactive approach helps mitigate the risks associated with viral contamination, safeguarding both patient safety and product integrity. Overall, plaque assays are indispensable analytical techniques in biomanufacturing, enabling precise quantification of viral contaminants and ensuring the production of safe and high-quality biopharmaceuticals.
TCID50 assay
The TCID50 (50% tissue culture infective dose) assay is vital for quantifying viral contaminants. Similar to plaque assays, TCID50 assays are essential for ensuring the safety and quality of biopharmaceutical products, including vaccines, recombinant proteins and gene therapies. TCID50 assays are particularly valuable for viruses that may not form distinct plaques, as they rely on the ability of the virus to cause cytopathic effects in cell cultures. This method allows biomanufacturers to accurately determine viral titers and assess the efficacy of viral clearance steps, contributing to the biopharmaceutical industry’s stringent regulatory compliance and product safety requirements.
Throughout biomanufacturing, TCID50 assays can monitor viral contamination levels at various stages, from raw materials to final products. These assays quantify viral loads in cell culture supernatants, viral vectors and other bioprocess intermediates, providing critical information for process control and optimization. Additionally, TCID50 assays are key in evaluating the effectiveness of viral clearance strategies, such as chromatography, filtration and inactivation methods, implemented during downstream processing. By quantifying the reduction in viral titers achieved by these purification steps, biomanufacturers can ensure the safety and purity of the biopharmaceutical products.
By routinely performing TCID50 assays on process intermediates and final products, manufacturers can verify the absence of viral contaminants and validate the reliability of their production processes. This proactive approach helps mitigate the risks associated with viral contamination, safeguarding patient safety and product quality. Overall, TCID50 assays are indispensable analytical techniques in biomanufacturing, enabling precise quantification of viral contaminants and ensuring the production of safe and effective biopharmaceuticals.
Enzyme-linked immunosorbent assay
Another method to detect viruses in the product process is enzyme-linked immunosorbent assay (ELISA). Here’s how ELISA works:
This type of ELISA starts with a microtiter plate coated with an antibody that binds to a specific antigen in a sample. The antigen is then “sandwiched” or binds to another antibody conjugated to an enzyme, catalyzing a chemical reaction that generates a detectable signal. That enzyme helps scientists ensure the virus is present because it can change color when another substance is added. If viral proteins are present, the enzyme attached to the second antibody reacts with this substance and causes the liquid in the well to change color.
The final step is to use a microplate reader to measure the intensity of the color change and quantify the amount of the virus present in the biopharmaceutical products.
ELISA can be a powerful tool in biopharmaceutical manufacturing because its sensitivity allows it to test many samples quickly and inexpensively. This helps scientists ensure that the products are safe and free from viral contamination.
Flow cytometry
Flow cytometry is a powerful tool used in biomanufacturing processes to quantify viruses, particularly for enveloped viruses or viruses labeled with fluorescent markers. This technique allows rapid and sensitive detection of viral particles by analyzing the fluorescence emitted by individual viral particles as they pass through a flow cytometer. In biopharmaceutical production, flow cytometry is often used for real-time monitoring of viral titers in cell culture supernatants, viral vectors and other bioprocess intermediates. By providing high-throughput and quantitative data on viral concentrations, flow cytometry lets biomanufacturers optimize production processes, assess viral infectivity and ensure the quality and safety of biopharmaceutical products.
Flow cytometry offers several advantages over traditional methods for virus quantification, such as plaque assays and TCID50 assays. Unlike these methods, flow cytometry does not rely on forming plaques or cytopathic effects in cell cultures, making it suitable for viruses that do not exhibit these characteristics. Additionally, flow cytometry allows for the simultaneous detection and characterization of viral subpopulations, providing valuable insights into viral heterogeneity and dynamics during bioprocessing. Flow cytometry represents a versatile and efficient approach for virus quantification in biomanufacturing, contributing to improved process control, product quality and regulatory compliance in the biopharmaceutical industry.
Mass spectrometry
Mass spectrometry is another powerful technique for detecting and identifying viruses in infected cells or biological samples. It analyzes the mass-to-charge ratios of peptides generated from viral proteins.
The process begins by collecting biopharmaceutical samples suspected of containing the virus. Depending on the analysis target, these samples are prepared to extract viral proteins or nucleic acids. Sample preparation methods may include protein extraction, nucleic acid isolation or digestion to generate peptides for analysis.
To be detectable by mass spectrometry, molecules in the sample must be given an electric charge that turns them into ions. After they are ionized, molecules are accelerated into a tube and separated by their mass-to-charge ratio. That happens because each molecule moves at a different speed based on its size and charge, with more highly charged ions moving more quickly.
A detector at the end of the tube records each ion that comes through, noting its speed and mass-to-charge ratio. Computers analyze that data, matching the mass-to-charge ratios of detected ions to known values for different viral proteins or peptides. When the mass-to-charge ratio of molecules in the tube matches that of a known virus, scientists know that viral contamination in the product must be addressed.
Current challenges in viral contamination
Viral detection is critical in biopharmaceutical manufacturing to help ensure the safety and efficacy of products, including vaccines or cell and gene therapies. Though many advancements have been made in viral detection and clearance, some challenges remain. They include:
- Sensitivity and specificity. Manufacturers must be able to detect low levels of viral contamination accurately. False positives could lead to unnecessary waste, delay and costs. False negatives could mean missing harmful contamination that risks patient safety.
- Emerging and unknown viruses. New viruses and variants may emerge that are not detectable with existing methods. Biopharma manufacturers must continuously develop new detection methods.
- Product complexity. Biopharmaceutical production is a complex process that uses living cells. That mix of cell proteins and other components can interfere with viral detection methods, making distinguishing between viral particle cell line components complex.
- Regulatory requirements. Guidelines for viral safety are stringent and constantly changing. Biopharma companies must ensure compliance by following the latest regulations and implementing new or updated testing methods.
- Sample preparation and processing. Accurate viral detection depends on good sample preparation and processing. Poor techniques could make viral particles undetectable.
- High-throughput screening. Rapid and precise screening methods are required to evaluate viral safety in many samples as biopharmaceutical production scales up. Manufacturers must devote the resources needed for automation and data analysis skills.
- Cost. Advanced viral detection methods that can detect many types of viruses can be expensive. Manufacturers must find the right balance between viral safety testing and the price of the technology.
- Cell and gene therapies. Cell and gene therapies can pose challenges for viral detection because viral vectors in these therapies can make it more difficult to detect viral contaminants.
Strategies for viral clearance
Virus clearance is a critical aspect of biomanufacturing processes and essential for ensuring the safety and quality of biopharmaceutical products. This process involves removing or inactivating viruses that may contaminate the manufacturing process and pose a risk to product safety.
Several strategies are employed to achieve virus clearance in biomanufacturing processes, including cell line selection, raw material screening and implementation of specific clearance steps. Choosing a cell line that is less susceptible to viral infection can reduce the risk of virus contamination. Cell lines with inherent resistance to common viruses or those that have been engineered to express viral resistance factors can be used. Raw materials, such as serum, media components and other additives used in biomanufacturing, should be screened for viruses before use.
Various steps within the biomanufacturing process, such as filtration, chromatography, chemical treatment and heat treatment, are designed to physically remove or chemically inactivate viruses, thereby minimizing the risk of viral contamination in biopharmaceutical products.
Viral inactivation
Virus inactivation methods are designed to deactivate or destroy viral contaminants while preserving the biological activity and efficacy of the product. Various virus inactivation strategies can be used, depending on the specific characteristics of the virus, the nature of the biopharmaceutical product or regulatory requirements. By implementing appropriate inactivation strategies tailored to the specific needs of the product and process, biomanufacturers can uphold stringent regulatory standards, safeguard patient health and maintain the integrity of the biopharmaceutical supply chain.
Chemical treatment is an effective method for virus clearance in biomanufacturing processes involving chemical agents to inactivate viruses by disrupting their structure or interfering with viral replication. Various chemical treatments can be applied at different stages of the manufacturing process to eliminate viral contaminants effectively. Common chemical treatments for virus clearance include using detergents, solvents and low-pH solutions. Detergents can disrupt the lipid envelope of certain viruses, rendering them noninfectious. Solvents, such as organic solvents or detergents, can disrupt viral membranes or capsids, leading to virus inactivation. Low-pH solutions, such as acid, can denature viral proteins and nucleic acids, effectively inhibiting viral replication.
Heat treatment techniques such as pasteurization, autoclaving or high-temperature short-time (HTST) treatment can expose the bioproduct to elevated temperatures for a specified duration. This effectively denatures viral proteins and nucleic acids and renders the viruses noninfectious. Other physical methods, such as irradiation with ultraviolet (UV) light or gamma radiation, can induce DNA damage or disrupt viral replication cycles, leading to viral inactivation.
Viral filtration
Viral filtration is a physical method of viral clearance that does not use chemicals that might change the product’s characteristics. It uses a specific filter with pore sizes designed to remove viruses from the process stream while allowing desired molecules to pass through. The filters have nanometer-sized pores that are too small for viruses to enter but are large enough for the product’s molecules to pass.
Biopharmaceutical products must be properly prepared before they are filtered. This involves removing any large particles or impurities that could clog filters. Prepared liquids can then be passed through the filter to remove viruses. Additional treatments or purification steps may be needed to help ensure the purity and potency of the product. Selecting virus filters depends on various factors, including the size and properties of the viruses of concern, the composition of the bioproduct and the desired level of virus removal. Virus filtration is often integrated into biomanufacturing processes as a dedicated virus clearance step, typically following other purification steps such as chromatography.
One challenge in viral filtration is to remove viruses without losing significant amounts of the product or reducing its efficacy. Scientists consider several factors, such as filter pore size, pressure and the volume of product, to maximize virus removal and minimize product loss.
Chromatography techniques
Chromatography can remove viral contaminants and purify biopharma products. This process works because different cells interact differently with a stationary phase — solid or liquid — and a mobile phase, such as a liquid or gas. Scientists can use this difference to separate viral cells from the rest of the product by specific characteristics such as size, charge, hydrophobicity or specific binding affinity.
Here are some of the chromatography techniques that can be used for viral clearance:
- Size exclusion chromatography (SEC), or gel filtration, separates virus cells from others based on size. The stationary phase consists of porous beads, with smaller molecules entering the pores and traveling a longer path while larger viral cells are blocked.
- Ion exchange chromatography separates viral from product cells by comparing their charge at a specific pH level. The stationary phase attracts cells with the opposite charge. By adjusting the pH and salt concentration of the mobile phase, scientists can control which cells are bound and which are eluted from the column to separate viruses and the product.
- Affinity chromatography is a clearance technique that binds specific target molecules to separate viruses from the product. The stationary phase, or solid support within the column, uses ligands with a strong affinity for target molecules in the product, such as proteins or antibodies, but not viruses.
- Hydrophobic interaction chromatography (HIC) separates molecules based on their hydrophobicity or ability to mix with water. HIC uses a hydrophobic stationary phase with high salt conditions. As the salt concentration decreases, viruses can be separated from product molecules because they have different levels of surface hydrophobicity.
Virus clearance validation
In bioprocessing, virus clearance validation is essential to guarantee the safety and purity of biopharmaceutical products. This process involves a rigorous assessment of the manufacturing steps to identify potential points of viral contamination and ensure effective clearance mechanisms. The validation begins with clearly defining the study objectives, including the specific viruses to be evaluated, desired clearance levels and acceptance criteria.
Next, representative model viruses that mimic potential contaminants that could compromise product safety are selected for spiking studies. These viruses are introduced at critical stages of the manufacturing process, allowing evaluation of clearance efficiency. Analytical methods such as PCR, plaque assays or virus-specific assays are employed to detect and quantify viruses throughout the process. Data collected from multiple validation runs are analyzed to assess the reduction or inactivation of viruses, ensuring compliance with predetermined acceptance criteria.
Finally, comprehensive documentation and reporting are essential to capture all experimental procedures, data and conclusions derived from the validation study. The report undergoes internal review and approval before being submitted to relevant stakeholders, including quality assurance and regulatory authorities. Continual monitoring is also established to periodically assess viral clearance during routine manufacturing operations, ensuring ongoing product safety and regulatory compliance
The regulatory landscape and compliance
Regulatory agencies aim to ensure that all biopharmaceutical products are safe and effective for patient use. This means the medicines must work as intended without harming patients, such as by exposing them to viral contamination.
Several agencies oversee biopharmaceutical production, the most prominent of which are the FDA and the European Medicines Agency (EMA). These agencies set standards and guidelines for viral safety in biopharmaceutical manufacturing that are followed worldwide.
Biopharmaceutical manufacturers must assess all possible sources of viral contamination before making the product. This includes examining raw materials, the cell lines used in production and the manufacturing process. They must develop tests to identify viral contamination at every stage.
Manufacturers must have methods in place to remove or inactivate any viruses that might be found in the product. These methods could include filtration, chromatography or chemical inactivation. Biopharma companies must keep detailed records to validate their viral detection and clearance processes. This can help prove to regulatory agencies that their methods are effective and consistent.
After developing biopharmaceutical products — including the necessary viral detection and clearance steps — the company must submit a detailed application to the regulatory agencies. The agencies review the application to ensure all safety measures are in place before approving the product for public use.
Complying with regulatory agencies does not end with approval. Biopharma companies must monitor production for safety on an ongoing basis, including tracking any potential viral contaminations. Any issues must be addressed promptly and reported to the regulatory agencies.
Protecting patient health and safety from viral contamination can be a complex process. It involves rigorous testing, specific viral clearance processes and thorough documentation to maintain regulatory compliance. Regulations and compliance are essential for maintaining the trust and safety of patients who use biopharmaceuticals for their health.
Future trends and innovation
New viral detection and clearance technology are creating a future in which biopharmaceuticals will be safer and more reliable than ever before. Here are some of the advancements and their potential impact:
- Next-generation sequencing (NGS) will allow scientists to quickly see a virus and read its genetic code by sequencing its DNA or RNA. NGS will also let scientists detect potential viral contamination more rapidly and detect viruses they did not know to look for.
- CRISPR is known as a gene-editing tool but can also be used as a precise virus detection tool. It can detect specific viruses from complex product mixtures. CRISPR technology could lead to very sensitive tests for viral contaminants, helping reduce false negatives.
- Advanced filtration techniques use new filter materials and nanotechnology to trap viruses more effectively. They could trap almost every virus based on size, shape or chemical properties, making the purification process more effective and less costly.
- Artificial intelligence and machine learning can help analyze large amounts of data to predict where viral contaminants might occur and suggest the best clearance methods. This could lead to more efficient manufacturing processes where viral contamination can be predicted and mitigated before it even happens.
- Broad-spectrum viral inactivation techniques include new chemical and physical methods to inactivate viruses without harming the therapeutic product. These methods include using light, pH changes or new chemicals that are more effective across a broader range of viruses.
The future impact
Integrating new technology into biopharmaceutical production promises to make viral detection and clearance more thorough, faster and cost-effective. This means that medicines can be produced with even higher safety standards and potentially lower costs, making them more accessible to patients worldwide.
As technology continues to evolve, we could see an increase in proactive prevention techniques that would make the risk of viral contamination in biopharmaceuticals almost nonexistent. Advanced technology and innovation can help move biopharmaceutical production toward a future where it is more efficient and medicines are safer.
A critical challenge
Detecting and removing viral contaminants from biopharmaceutical products is a critical challenge that requires precision, advanced technology and strict adherence to regulatory standards.
Continuous and robust viral detection and clearance protocols are essential to protect public health, safeguard the integrity of the products and comply with regulatory demands.
Emerging technology, such as NGS, CRISPR-based detection systems and advanced filtration methods, represent the forefront of innovation and will help enhance viral clearance protocols’ efficiency, accuracy and cost-effectiveness.
Regulatory agencies will continue to play a crucial role in maintaining high safety and efficacy standards to protect patient safety. Their stringent guidelines help ensure manufacturing processes adhere to quality standards, building trust in the safety of biopharmaceutical products.
Artificial intelligence, machine learning and broad-spectrum viral inactivation techniques will enhance biopharmaceutical production by refining detection and clearance methods and allowing scientists to identify and mitigate potential viral contamination preemptively. Every technological advancement helps bring the industry closer to a future in which medicines’ safety and efficacy reach unprecedented levels.
Here is a list of essential tools available from Avantor for viral detection.
Learn more about viral clearance by watching Enhancing Virus Clearance Process Capability Using a Biodegradable Detergent.
Learn about new approaches to viral inaction by watching Novel Approach to Purification and Viral Inactivation of new class of mAbs.