Cell Therapy Manufacturing
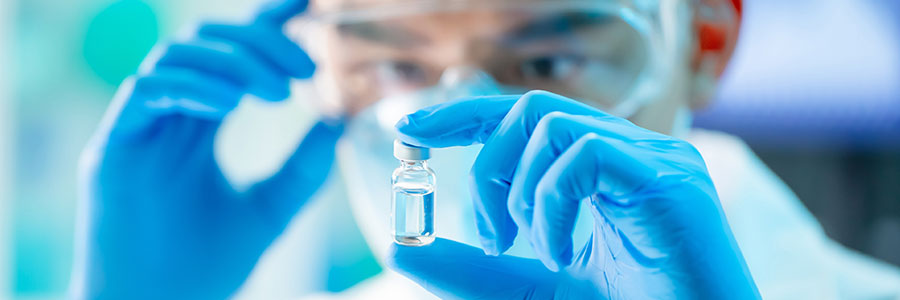
Cell therapy is an advanced biomedical research and treatment area in which physicians administer live cells into a patient’s body. Among the applications of cell therapy are treating cancers and autoimmune diseases or regenerative medicine for repairing damaged tissues.
Cell therapy manufacturing involves taking cells from the patient (autologous) or a donor (allogeneic). In addition to sourcing cells, the process includes isolating, culturing and modifying cells in a laboratory to prepare them to treat a wide range of medical conditions.
What is cell therapy used for?
Cell therapy is an innovative approach to modern medicine that offers new treatment solutions for a variety of illnesses and medical conditions. Here are some current and potential uses for cell therapy:
Regenerative medicine
- Tissue regeneration and repair. Cell therapy is used in regenerative medicine to repair or replace damaged tissue and organs. Stem cells, such as mesenchymal stem cells (MSCs), can regenerate bone, cartilage, and muscle tissues. This helps physicians treat injuries and degenerative diseases.
- Cardiovascular disease. Stem cells can be used to repair heart tissues damaged by myocardial infarction (heart attack) or heart failure by helping to regenerate heart muscle to improve heart function.
Oncology (cancer treatment)
Some of the most groundbreaking cell therapy applications are in oncology, where it can treat cancers such as leukemia and lymphoma.
- CAR T cell therapy. Chimeric antigen receptor T cell (CAR-T) therapy uses a patient’s modified T cells to express a receptor that targets and destroys cancer cells.
- Tumor-infiltrating lymphocytes (TILs). This approach involves extracting immune cells from a patient’s tumor, expanding them in the lab and reintroducing them into the patient to boost the patient’s immune response against cancer.
Autoimmune diseases
- Stem cell transplants. Hematopoietic stem cell transplantation (HSCT) can treat autoimmune diseases such as multiple sclerosis (MS) and systemic lupus erythematosus (SLE) by using cell therapy to reset a patient’s immune system to halt disease progression.
- Regulatory T cells (Tregs). Scientists are studying Tregs because they have the potential to modulate the immune system and treat autoimmune conditions by suppressing overactive immune responses.
Neurological disorders
- Parkinson’s disease. Cell therapy is being investigated for its potential to replace lost or damaged neurons in patients who have Parkinson’s disease. Dopamine-producing cells derived from stem cells may be transplanted to restore dopamine levels and improve motor function.
- Spinal cord injuries. Researchers are studying stem cells to learn more about their ability to regenerate damaged spinal cord tissue and restore motor and sensory functions in patients with spinal cord injuries.
Orthopedic applications
- Osteoarthritis. Mesenchymal stem cells (MSCs) can treat osteoarthritis by repairing and regenerating cartilage, reducing pain and improving joint function.
- Bone fractures. Cell therapy can enhance bone healing in complex fractures by promoting new bone formation and reducing healing time.
Skin disorders and wound healing
- Chronic wounds. Stem cells can treat chronic wounds, such as diabetic ulcers and pressure sores by promoting tissue regeneration and improving wound closure.
- Burns. Cell therapy can accelerate burn wound healing by regenerating skin tissue and reducing scar formation.
Ophthalmology
- Corneal repair. Ophthalmologists can use stem cells to repair damaged corneas and restore vision in patients with corneal injuries or diseases.
- Retinal disease. Research is underway to learn how stem cells could regenerate retinal cells to treat retinal diseases like macular degeneration and retinitis pigmentosa and restore vision.
Type 1 diabetes
Scientists are investigating cell therapy to replace the insulin-producing beta cells destroyed by the immune system in patients with Type 1 diabetes. Stem cells can be differentiated into beta cells and transplanted to restore insulin production.
How are cell therapies made?
Cell therapy manufacturing involves several critical steps that ensure their safety and effectiveness. In some cases, genetic modifications enhance the therapeutic abilities of the cells by using techniques such as gene editing. Strict quality control measures are used at each stage of this process to ensure the cells are safe and have the correct identity and functionality.
The manufacturing process for cell therapies includes five steps. They are:
- Cell sourcing and isolation. Cells can be sourced from patients or donors, and the type of cell used depends on the therapy. For example, hematopoietic stem cells are often used to treat blood disorders. Cells are isolated from the source material, such as blood, bone marrow or tissue samples. This step requires accuracy to harvest the correct cell types.
- Cell culture and expansion. After they are isolated, cells can be cultured in a lab using growth media and conditions. The cells may be genetically modified to target specific diseases or enhance their efficacy in this phase.
- Quality control and characterization. Cells are subjected to rigorous quality control tests throughout the manufacturing process. These tests are designed to ensure the cells are viable, are contaminant-free and have the desired characteristics. Characterization techniques include flow cytometry, genetic analysis and functional assays.
- Cryopreservation. Cells are often cryopreserved for storage and transport. This involves freezing the cells at ultra-low temperatures to maintain their viability until they are needed for therapy.
- Final formulation and packaging. The final step of the manufacturing process is to formulate the cells into a product that can be administered to patients. This includes ensuring the correct cell concentration and packaging the product in sterile, ready-to-use formats.
Regulatory and compliance
Cell therapy manufacturing is heavily regulated to ensure patient safety and product efficacy. Regulatory bodies such as the U.S. Food and Drug Administration (FDA) and the European Medicines Agency (EMA) have strict guidelines for producing, testing and using cell-based therapies. Complying with Good Manufacturing Practices (GMP) is essential and requires meticulous documentation and validation during each manufacturing process step.
Cell therapy manufacturing techniques
Cell therapy manufacturing includes sophisticated techniques and processes for producing innovative therapeutic approaches. It involves exploring diverse cell sources — autologous or allogeneic — to find the correct starting material for each therapy. Cell isolation and expansion steps create a strong foundation for building therapeutic cell populations.
Exploring different cell sources for therapy production
Cell therapy offers promising treatments for many conditions and traumas. Choosing a suitable cell source is critical for achieving positive outcomes in cell therapies because it affects safety, efficacy and ethical issues. Different cell sources can be used in therapy development, and each cell has specific benefits and challenges.
Embryonic stem cells (ESCs): These pluripotent cells are derived from embryos and can differentiate into any cell type in the body. Because of their origin, ethical and immunological concerns about their use exist.
Induced pluripotent stem cells (iPSCs): iPSCs are created by reprogramming adult cells to a pluripotent state that can differentiate into different cell types. That means iPSC cells offer the versatility of ESCs without ethical concerns.
Adult stem cells: Adult stem cells are found in various tissues and organs and can differentiate into specific cell types. They are readily available but may have limited differentiation potential compared to pluripotent cells.
Mesenchymal stem cells (MSCs): MSCs are versatile cells found in sources like bone marrow and adipose tissue. Their immunomodulatory properties allow them to treat inflammatory conditions.
Cell isolation and expansion methods: A step-by-step guide
Cell isolation and expansion require a carefully structured process. It begins with preparing a suitable culture medium that provides the nutrients and environment needed for cell growth. Optimizing other cell growth conditions, such as temperature and oxygen levels, promotes cell proliferation while maintaining quality. Continuous monitoring and interventions are essential throughout this process to ensure cell viability and functionality and produce healthy and functional therapeutic cells.
Preparing the culture medium
Creating an appropriate culture medium is a vital step in the isolation and expansion of cells. This specialized medium helps cells thrive and significantly impacts their well-being and growth.
The culture medium must supply essential nutrients, ions and growth factors for cells to survive and multiply. This typically includes sugars (like glucose), amino acids, vitamins, salts and minerals. The medium's pH level and osmolarity should be regulated to maintain a stable and favorable setting for cell growth.
Culture medium selection varies depending on the specific type of cells and intended application. Some cells may require specialized media with growth factors or supplements that encourage differentiation or maintain particular characteristics. The culture medium must be sterile to prevent contamination that could jeopardize cell health and the reliability of research outcomes.
Optimizing cell growth conditions
Several factors, including temperature, oxygen levels and nutrient provision, can significantly impact cell proliferation and quality. Adjusting these conditions can create an ideal environment for growth and high-quality cultures.
Temperature: A temperature of 37 C is optimal for most mammalian cells, but microbial cultures may require different temperatures. Regulating temperatures is essential to supporting the regular activity of enzymes and metabolic processes.
Oxygen levels: The oxygen levels within the culture must closely match the physiological requirements of cells. Aerobic bacteria thrive in environments with high oxygen levels, while anaerobic bacteria or specific types of stem cells prefer lower oxygen levels (hypoxic conditions).
Nutrient supply: The culture media must strike the right balance regarding sugars, amino acids, vitamins and minerals to maintain proper nutrient supply. Changing the media regularly helps replenish essential nutrients and eliminates waste products so the cells can grow in optimum conditions.
pH and osmolarity: Maintaining precise pH and osmolarity levels is vital to maintaining cellular equilibrium. Cells exhibit sensitivity to fluctuations in these parameters, and any deviations can significantly impact growth and viability.
Monitoring and maintaining cell viability and functionality
Consistently monitoring cell viability helps produce functional therapeutic cells by helping to maintain cell health and preserve desired characteristics. These steps can help ensure the production of robust, functional cells for various applications.
Continuous evaluation: The ongoing assessment of cell viability and growth using techniques such as cell counting, microscopy and viability assays can be an early warning system for detecting signs of stress or contamination.
Controlled environment: Creating and maintaining a precisely controlled environment, including temperature, humidity and gas levels (CO2 and O2), is essential to preserving cell health and functionality.
Nutrient and waste management: A regular schedule of culture media replacement helps provide a consistent supply of vital nutrients and removes waste products.
Stringent quality control: Rigorous quality control procedures to confirm cell identity, purity and functionality at different stages of the culture process can protect against unwanted alterations or deviations.
Quality control and assurance in cell therapy manufacturing
Quality control and assurance are important in cell therapy manufacturing because they help ensure safety and efficacy. This involves establishing cell identity and functionality through rigorous testing and validation. It also focuses on maintaining sterility and safety by following regulatory guidelines and Good Manufacturing Practices. Quality management systems help manage every stage of the production process, from cell sourcing to final formulation, and emphasize consistency, traceability and regulatory compliance.
Establishing the identity and functionality of cells
Establishing cell identity and functionality is critical for the success and safety of cell therapy. It includes using precise methodologies and assessments for cell surface marker analysis, genetic profiling and functional assays.
Genetic profiling studies gene expression patterns to help ensure the cells carry desired characteristics. Functional assays evaluate the cell’s capacity to perform specific functions or tasks to help confirm their therapeutic potential. Together, these tests can comprehensively evaluate the cells and confirm they have the essential attributes for therapeutic applications.
Ensuring sterility and safety through testing and validation
Maintaining sterility and safety in cell therapy products helps safeguard patient health and product quality. That requires creating and implementing a comprehensive slate of environmental monitoring, raw material testing and a combination of in-process and end-product testing. Consistent process and product validation help ensure cell therapy products are safe, effective, and compliant with regulatory requirements.
Implementing a quality management system
A quality management system (QMS) can help ensure high-quality cell therapy products are produced according to established standards. A QMS can include policies, procedures and protocols that govern every stage of the production process.
Quality management systems help standardize the manufacturing process, from cell acquisition and cultivation through formulation and final product release. They involve thorough documentation, traceability and continuous monitoring to reduce the chance of errors and deviations.
Key challenges in cell therapy manufacturing
Cell therapy manufacturing faces several challenges. One challenge is scaling up production to meet the growing demand for these therapies while maintaining product quality. Another is standardizing processes to help ensure consistent and reproducible results. Quality control and regulatory compliance are additional challenges.
Scalability
Expanding cell therapy production from laboratory scale to clinical and commercial scale while maintaining cell quality can be difficult. A successful transition requires comprehensive planning, sufficient investment and creative solutions to any problems that might occur.
Standardization
Standardized protocols are necessary for consistency among several batches of cells and across different production sites. Standardization includes establishing validated systems to help ensure that processes are reliable and repeatable and control other variables. That’s why sourcing, culture mediums and cell products are consistent for the safety and effectiveness of cell therapies, obtaining regulatory approvals, and cultivating trust among healthcare professionals and patients.
Cost
Consistent, high-quality cell therapy manufacturing can be costly because of the complex and time-consuming processes involved. Addressing that cost requires several approaches, including technological innovation, process optimization and strategic collaborations.
New developments in automation, bioreacavanttor technology and process optimization address these challenges. Single-use technology, for example, streamlines manufacturing workflows and reduces the risk of contamination. Artificial intelligence and machine learning enhance process control and quality assurance in cell therapy manufacturing.
Advances in cell therapy manufacturing
Significant advancements in cell therapy manufacturing are continuing to reshape the field of regenerative medicine. For example, researchers use advanced methods such as CRISPR-Cas9 to modify cell genes to enhance their therapeutic capabilities accurately. This allows the creation of more powerful and specifically directed cell therapies that could cure a wider variety of diseases efficiently.
Progress also includes the ongoing introduction of new tools and technologies into the cell therapy manufacturing process. Automation, robotics, and machine learning have helped simplify the manufacturing workflow to increase reliability and scalability. This not only reduces production expenses but also helps improve the quality and safety of the final products. New bioreactor systems and sophisticated three-dimensional (3D) culture techniques are becoming increasingly important for the mass production of therapeutic cells.
Avantor® and cell therapy manufacturing
Avantor provides comprehensive solutions to support the cell therapy manufacturing process. Our products and services include high-quality cell culture media, reagents and equipment designed to meet the specific needs of cell therapy production. Avantor is committed to advancing the field of cell therapy and ensuring that these life-changing treatments reach patients safely and efficiently.
For more information on Avantor’s cell therapy products research and manufacturing solutions, visit our website or contact our customer service team.