Cell Culture Media Types
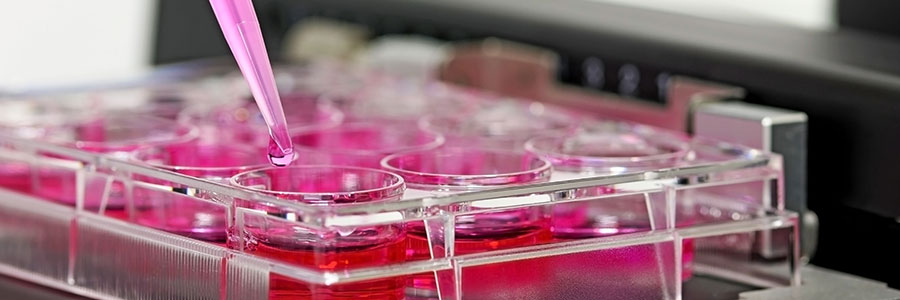
Cell culture media play a vital role in growing cells outside their natural surroundings for research or industrial applications. These nutrient-rich solutions support cell growth by supplying essential nutrients, hormones and growth factors. There are different types of cell culture media designed to meet specific cell requirements, such as serum-containing media that cater to diverse needs and serum-free media to minimize variability.
Defined media offer precise control over components, while specialized media are tailored for specific cell types. The choice of media depends on the desired outcomes and the characteristics of the cells involved. Selecting the right media is crucial, as it significantly influences cell behavior and experimental results, particularly in fields like biotechnology and medicine.
Classification of cell culture media types
Cell culture media types can be broadly categorized into serum-based, serum-free and chemically defined types. Serum-based media contain animal sera, providing a rich mix of growth factors but posing challenges of variability and ethical concerns. Serum-free media exclude animal-derived components, offering better reproducibility and reduced contamination risk but demanding meticulous optimization.
Chemically defined media use precisely defined components to ensure standardized conditions with advantages of reproducibility and control, but they also lack unidentified factors present in sera. h type presents distinct advantages and challenges, catering to diverse applications in biopharmaceuticals, research and biotechnology.
Serum-Based Media
Serum-based cell culture media is a specialized type of cell culture medium used in laboratory settings for the in vitro cultivation of cells. It contains serum, the liquid portion of blood without clotting factors, which is crucial for providing essential nutrients, growth factors, hormones and other elements necessary for cell growth and proliferation. Fetal bovine serum (FBS) is commonly chosen for its rich nutrient content.
The components of serum-based media include the following:
Serum: Serum is a critical component and provides essential nutrients, growth factors, hormones and other factors necessary for cell growth and proliferation. Example: Fetal bovine serum.
Nutrients: These include sugars, amino acids, vitamins and minerals that support cellular metabolism and growth.
Buffers: Maintaining the pH of the media is crucial for cell viability. Buffers help regulate and stabilize the pH levels.
Salts: Inorganic salts provide ions necessary for various cellular functions, including enzyme activity and osmotic balance.
Cytokines and Growth Factors: These are specialized signaling molecules found in the serum. They play a crucial role in controlling various aspects of cell behavior, including differentiation, proliferation and survival.
Trace Elements: Essential metals like iron, zinc and copper are included in trace amounts to support enzymatic activities.
Using serum-based media comes with various advantages and disadvantages that are listed below:
Advantages of Using Serum-Based Media
Rich Nutrient Content: Serum provides a broad spectrum of nutrients that support cell growth, making it suitable for a wide range of cell types.
Complex Mix of Growth Factors: The presence of growth factors in serum aids in cell proliferation and differentiation, mimicking the in vivo environment.
Established Protocols: There are well-established protocols for using serum-based media, making it a reliable choice with a history of success in various cell culture applications.
Disadvantages of Using Serum-Based Media
Batch Variability: Serum composition can vary between batches, leading to inconsistencies in cell culture conditions.
Risk of Contaminants: Serum may contain contaminants, such as viruses, mycoplasma or prions, which can negatively impact cell cultures. However, employing filtration techniques can minimize the risk of contamination, such as those offered by Seradigm.
Ethical and Cost Considerations: The collection of serum, especially fetal bovine serum, raises ethical concerns, and it can be expensive.
Serum-Free Media
Serum-free media refers to cell culture media that do not contain serum. These media are designed to support the growth and maintenance of cells in vitro without the need for serum supplementation. The transition from serum-containing to serum-free media has been driven by the desire for more defined and reproducible cell culture conditions. The composition of serum-free media involves the following:
Basal Medium: Serum-free media typically start with a basal medium that provides essential nutrients and ions required for cell growth. Common basal media include DMEM (Dulbecco’s Modified Eagle Medium) or RPMI (Roswell Park Memorial Institute) medium.
Nutrient Supplements: Essential nutrients such as amino acids, vitamins and minerals are added to mimic the physiological environment and support cell metabolic activities.
Hormones and Growth Factors: Serum-free media often incorporate specific hormones (e.g., insulin) and growth factors (e.g., epidermal growth factor) to regulate cell signaling pathways and promote cell proliferation.
Trace Elements: Trace elements like selenium and copper are included in precise concentrations to meet cellular requirements.
Buffering Agents: To maintain a stable pH, buffering agents like HEPES or bicarbonate are commonly used.
Antibiotics and Antimycotics: These may be added to prevent bacterial and fungal contamination during cell culture. It is a common practice to include antibiotics and antifungals to maintain a sterile environment. However, in primary cell cultures or specialized experiments, researchers may choose not to use them.
Using serum-free media comes with various benefits and challenges that are listed below:
Benefits of Serum-Free Media
Defined Composition: Serum-free media offer a precisely defined composition, reducing variability between experiments and enhancing reproducibility.
Reduced Batch-to-Batch Variability: The absence of serum eliminates the batch-to-batch variability associated with serum-containing media, providing a more consistent environment for cell growth.
Avoidance of Contaminants: Serum-free conditions decrease the risk of introducing contaminants present in serum, such as viruses, prions or undefined growth factors.
Controlled Experimental Conditions: Researchers have better control over experimental conditions to study specific cellular responses and signaling pathways.
Challenges of Using Serum-Free Media
Optimization: Serum-free media require optimization for specific cell types, and finding the right combination of supplements can be time-consuming.
Cell Adaptation: Some cell lines may take time to adapt to serum-free conditions, impacting cell viability and growth initially.
Cost: Serum-free media can be more expensive than traditional media-containing serum, which may be a consideration for large-scale cell culture.
Specialized Requirements: Certain cell types may have unique requirements that are more easily met with the complexity of serum-containing media.
Serum-free media offer a defined and controlled environment for cell culture, with benefits in terms of reproducibility and reduced variability. However, overcoming challenges such as optimization and adaptation is essential for successful implementation in specific experimental contexts.
Chemically Defined Media
Chemically defined cell culture media refers to a type of growth medium used in microbiology and cell culture. It is precisely formulated with known quantities of all the individual components. This is in contrast to complex media, which contain undefined components such as extracts, peptones or other complex substances of unknown composition.
The formulation of chemically defined a media is carefully designed to provide all the essential nutrients required for the growth of specific microorganisms or cells. Chemically defined media involve the following ingredients and properties.
Amino Acids: Chemically defined media typically contain a defined set of amino acids. This ensures that the cells have access to all the necessary building blocks for protein synthesis.
Sugars/Carbon Sources: Specific carbon sources, such as glucose, are included to provide energy for cellular processes.
Vitamins and Minerals: Essential vitamins and minerals are included in precise amounts to support various metabolic pathways.
Salts: Inorganic salts, such as phosphates and sulfates, are added to maintain osmotic balance and provide essential ions.
Trace Elements: Some media include trace elements like iron, zinc and copper in defined concentrations.
pH Buffers: Buffers are often incorporated to maintain a stable pH, crucial for enzymatic activity and cell function.
Chemically defined media has several applications that make it a crucial media type in microbiology and cell culture.
Applications
Research and Development: Chemically defined media are widely used in research settings, allowing scientists to control and manipulate specific variables in experiments.
Bioprocessing: In the biotechnology industry, chemically defined media play a crucial role in the production of therapeutic proteins and other biopharmaceuticals.
Cell Culture: In cell culture applications, chemically defined media are used to cultivate cells for various purposes, such as vaccine production or tissue engineering.
However, despite its obvious benefits and applications, chemically defined media suffer from certain limitations and setbacks.
Limitations
Complexity and Cost: Formulating chemically defined media can be complex, and the cost of producing such media is often higher than complex media.
Specificity: Chemically defined media are often tailored to the requirements of specific organisms or cell lines. The need for customization limits their broad applicability.
Cell Adaptation: Some cells may not readily adapt to chemically defined media, requiring optimization and adaptation periods.
Chemically defined media provide a controlled environment for microbial or cellular growth by offering a precisely defined composition. While they find extensive use in research and bioprocessing, their customization and cost pose challenges that must be considered in their application.
Optimizing cell culture media
Cell culture can be optimized using a two-pronged approach. First, by diving deep into biochemistry, using advanced methods to analyze things like nutrient levels, pH, osmolality and potential contaminants. Second, we could focus on improving various elements of the cell culture process. This might involve adjusting temperature and agitation, fine-tuning the composition of the cell culture medium or using advanced monitoring and control systems to ensure the best possible conditions.
This dynamic process ensures that we create a customized and consistent environment for cell growth, promoting strong and reliable experiment results. Combining thorough analysis and strategic adjustments makes optimizing cell culture media a crucial aspect of biopharmaceuticals, research and biotechnology.
Biochemical Analysis of Cell Culture Media
The biochemical analysis of cell culture media is a critical aspect of ensuring optimal conditions for cell growth and function. This analysis is carried out through methods for analyzing nutrient content, such as quantitative analysis, qualitative analysis, biosensors and metabolomics.
Methods for Analyzing Nutrient Content
1. Quantitative Analysis
Spectrophotometry: It is a widely employed technique in quantitative analysis, particularly for measuring concentrations of specific nutrients in various samples. This method relies on the principle that different compounds absorb light at characteristic wavelengths. By assessing the absorbance of light at these specific wavelengths, scientists can determine the concentration of a particular substance in a sample.
High-Performance Liquid Chromatography (HPLC): This is a powerful analytical technique that allows for the separation and quantification of individual components within complex mixtures. In HPLC, a liquid sample is passed through a column packed with a stationary phase, and the various components are separated based on their interactions with this phase.
Mass Spectrometry: It is a versatile technique used for the identification and quantification of various compounds in a sample based on their mass-to-charge ratio. This method is crucial in fields such as proteomics, metabolomics and environmental analysis.
2. Qualitative Analysis
Liquid Chromatography-Mass Spectrometry (LC-MS): LC-MS combines the capabilities of liquid chromatography and mass spectrometry, offering a powerful tool for qualitative analysis. This method is particularly useful for identifying and characterizing components in complex samples, such as biological fluids or environmental extracts.
Gas Chromatography (GC): Gas Chromatography is a technique suitable for the separation and analysis of volatile compounds. In GC, a sample is vaporized and passed through a column, where its components are separated based on their affinity for the stationary phase.
3. Biosensors
Biosensors are analytical devices that utilize biological components to detect and quantify specific substances. Fluorescent probes are a type of biosensor that provides real-time monitoring of nutrient levels. These probes emit fluorescence in the presence of the target nutrient, allowing for continuous and sensitive measurement.
Moreover, Cedex and YSI systems are also widely employed to evaluate amino acids, metabolites and waste products using methods like impedance measurements or enzymatic reactions. While biosensors like fluorescent probes provide immediate insights, alternative tools such as Cedex and YSI systems contribute to a thorough understanding of cell cultures and fermentation processes. They offer accurate data on vital parameters like cell density, viability and metabolite concentrations.
Enzyme-linked assays are another biosensing approach involving specific enzymes linked to nutrient reactions. When the nutrient of interest is present, the enzyme produces a detectable signal, enabling precise and selective detection.
4. Metabolomics
Metabolomics involves the comprehensive study of small molecules, or metabolites, present in biological systems. Nuclear Magnetic Resonance (NMR) is a nondestructive analytical technique used in metabolomics to provide detailed information about the structure and concentration of metabolites. Metabolite profiling is a broader approach in metabolomics that involves examining the overall metabolic fingerprint of a sample, providing insights into the physiological state of an organism or the composition of a complex mixture.
Importance of Monitoring pH, Osmolality and Contamination Level
1. pH
pH plays a pivotal role in various biological processes. It is a critical determinant influencing enzyme activity and metabolic reactions in cell metabolism. Cells maintain a specific pH range, typically around 7.4, to ensure optimal conditions for biochemical pathways, facilitating cell viability. Furthermore, pH intricately governs protein stability and structure, crucial for the proper functioning of cellular components.
2. Osmolality
Osmolality is integral to cellular homeostasis and guarantees the proper balance of water and solutes within cells, influencing their overall health and functionality. It directly affects cell volume and plays a key role in preventing excessive swelling or shrinkage. Maintaining the right osmolality is essential for ensuring that cells function optimally and their structural integrity is preserved.
3. Contamination Level
Contamination in cell cultures poses a significant threat to both cell viability and the reliability of experimental outcomes. Microbial contamination, whether bacterial, fungal or viral, can infiltrate cell cultures, jeopardizing the health of cells and potentially skewing experimental results. The impact extends beyond mere cell viability; it influences data reproducibility as well.
4. Quality Assurance
Quality assurance in cell culture involves meticulously monitoring various parameters to ensure consistent and controlled conditions. This consistency is crucial for promoting optimal cell growth and maintaining a stable environment for experiments. By upholding specific standards in pH, osmolality and preventing contamination, quality assurance measures guarantee that experimental conditions remain reliable and reproducible.
Strategies for Media Optimization
Media optimization is a critical aspect of cultivating cells or microorganisms for various purposes, such as biopharmaceutical production, biofuel development or basic research. It involves refining the composition of the growth medium to enhance the growth and productivity of the target organisms. Two fundamental strategies for media optimization include adjusting nutrient concentrations and ratios and screening and optimizing growth factors and supplements.
Adjusting Nutrient Concentrations and Ratio
Optimizing Nutrient Levels: Regularly assess and adjust the concentrations of key nutrients based on the specific requirements of the cells in culture. This may involve titrating the levels of amino acids, glucose, salts and other essential components.
Balancing Nutrient Ratios: Consider the stoichiometry of nutrient requirements. Cells often have specific ratios of nutrients they need for optimal growth. Adjusting these ratios can influence metabolic pathways and improve overall performance.
Monitoring Metabolites: Keep a close eye on metabolic by-products. Accumulation of waste products can inhibit cell growth. Adjusting nutrient levels can help mitigate the build-up of undesirable metabolites.
Screening and Optimizing Growth Factors and Supplements
Identification of Key Growth Factors: Understand the specific growth factors required for the cell type in culture. This may involve conducting screening experiments to identify the most effective combinations and concentrations.
Fine-Tuning Concentrations: Once identified, optimize the concentrations of growth factors to achieve the desired cellular responses. Too much or too little of a growth factor can have detrimental effects on cell behavior.
Supplementing With Extracellular Matrix Components: In certain cell cultures, adding extracellular matrix components or mimetics can improve cell adhesion, migration and differentiation. Experiment with different matrix supplements to find the most suitable for the desired outcomes.
By carefully adjusting the nutrient concentration, screening/optimizing growth factors and supplements, researchers and biotechnologists can tailor the culture media to the specific needs of the cells, leading to improved growth, viability and productivity in various applications, including bioprocessing and tissue engineering.
Future perspectives on cell culture media types
Dynamic trends and innovations mark research in cell culture media types. Cutting-edge technologies, advanced formulations and novel methodologies are shaping the landscape to improve reproducibility, reduce variability and optimize conditions for various cell types. Researchers are pushing boundaries by integrating synthetic components, exploring serum-free and chemically defined media and striving to meet the evolving needs of biopharmaceuticals, regenerative medicine and basic research.
A notable trend is the development of personalized media types, where researchers tailor formulations to specific cell types or applications. This customization aims to optimize outcomes in biopharmaceutical production and research by precisely controlling growth conditions. This approach enhances cell viability, productivity and the reproducibility of experiments, reflecting a paradigm shift toward more targeted and efficient methodologies in cell-based research.
Despite the promising strides in personalized cell culture media types, there are challenges to address. Scalability issues, regulatory compliance complexities and media formulation suitable for diverse cell types pose significant hurdles. However, these challenges also present opportunities for breakthroughs in biotechnological advancements.
Innovations in scalable, defined and regulatory-compliant media formulations have the potential to revolutionize bioprocessing, personalized medicine and therapeutic applications. Striking a balance between addressing challenges and leveraging opportunities is crucial for advancing the field and meeting the demands of modern cell culture applications.
Conclusion
Cell culture media are crucial for cultivating cells in vitro, influencing cell growth and behavior. These media are classified into three types: serum-based, serum-free and chemically defined media. Optimizing media involves meticulous biochemical analysis, considering nutrient content and monitoring critical factors like pH and osmolality. Strategic adjustment of nutrient concentrations and screening growth factors are pivotal strategies.
Looking ahead, the field anticipates personalized media formulations and addresses challenges in novel formulations. Advances in cell culture media types highlight their pivotal role in advancing research and biotechnology, offering a glimpse into a future where tailored formulations enhance cell culture precision and efficacy.
You can elevate your cell culture experiments with Avantor and achieve optimal cell growth and performance with sera grades from Seradigm. Through impeccable research and quality assurance, our range of cell culture media ensures reproducibility and reliability in your experiments.