Strategies for Optimizing Downstream Processing
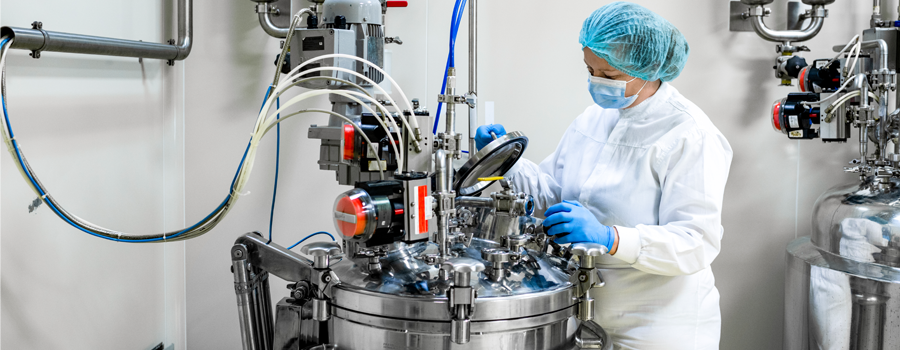
Downstream processing is vital for efficient and cost-effective production of biopharmaceuticals, industrial enzymes and other bio-based products. Downstream processing refers to purifying, separating and recovering the desired product from the complex mixture obtained after upstream processes like fermentation or cell culture.
With the right strategies for improving downstream processing, you can enhance the efficiency, productivity and quality of your downstream process. We will discuss advanced chromatography techniques like affinity chromatography, ion exchange chromatography, hydrophobic interaction chromatography and size exclusion chromatography for obtaining high-purity product recovery. Additionally, we will highlight the importance of process optimization, including process parameter optimization, unit operations and purification step integration.
The importance of downstream processing
Downstream processing plays an important role in various industries, especially biotechnology and pharmaceuticals. Its primary goal is to separate and purify the desired product from the complex mixture of substances generated during upstream processes, such as fermentation or cell culture.
It plays a vital role in industries reliant on biological products, ensuring product purity, optimum yield, quality control measures, and safety and regulatory compliance, and achieving cost-effectiveness. Additionally, efficient downstream processing strategies enable companies to obtain high-quality products that meet regulatory standards while maximizing production capabilities. The significance of downstream processing can be attributed to several key factors:
Product Purification
Downstream processing allows for the purification of the desired product to meet specific quality and regulatory standards. It involves removing impurities, such as host cell proteins, DNA, endotoxins, adventitious viruses and other contaminants that may be present in the crude mixture. Purification ensures the safety, efficacy and consistency of the final product.
Yield Optimization
Efficient downstream processing techniques can maximize product recovery and yield. This is critical for industries where the target product is scarce or expensive to produce. By employing suitable separation and purification strategies, the overall process efficiency can be improved, leading to higher product yields.
Quality Control
Downstream processing facilitates quality control by enabling the analysis and characterization of the final product. Various analytical techniques, such as high-performance liquid chromatography, electrophoresis, mass spectrometry and spectroscopy, and enzyme-linked immunosorbent assay (ELISA), are employed to assess the purity, identity and activity of the product. These tests ensure that the product meets the desired specifications and is safe for use.
Safety and Regulatory Compliance
Downstream processing plays a crucial role in ensuring product safety and complying with regulatory guidelines. It involves the removal of potential contaminants, including pathogens, toxins and impurities, which could pose risks to human health. Compliance with regulatory standards is necessary to obtain approvals from regulatory authorities before commercialization.
Scalability and Cost-Effectiveness
Downstream processing techniques need to be scalable to meet the demands of large-scale production. The development of robust and cost-effective downstream processes is essential to make the final product economically viable. Optimization of process parameters, selection of appropriate purification techniques and implementation of efficient separation methods contribute to cost reduction.
Downstream Processing Harvesting Techniques
Harvesting is a crucial step in downstream processing that involves collecting the desired product from the bioreactor or culture medium. To efficiently separate the target product, a range of techniques are employed. These include centrifugation, filtration, flocculation, foam fractionation and precipitation. Researchers and industrial practitioners utilize these methods to effectively recover and concentrate the desired product, facilitating subsequent purification and formulation processes.
However, it’s important to consider that the choice of harvesting technique depends on various factors. These factors include the nature of the product, the type of cells or biomass involved, the scale of production and the downstream processes planned for implementation. To achieve optimal separation and recovery, different combinations of these techniques can be employed in a sequential manner. In the field of biopharmaceuticals, several common harvesting techniques are utilized.
Centrifugation
Centrifugation is a widely used technique that separates components in a mixture based on their differences in density and size. It involves subjecting cells or particles to high-speed rotation, resulting in the formation of a pellet and a supernatant. The pellet contains impurities, while the supernatant typically contains the desired product. However, when it comes to harvesting monoclonal antibodies (mAbs), the target product is found in the supernatant rather than the pellet.
During the production of mAbs, the producing cells, often mammalian cells in a bioreactor, secrete the antibodies into the surrounding nutrient-rich medium. Therefore, when it’s time to harvest the mAbs, the supernatant, which is the liquid portion of the mixture, is collected. This supernatant contains the valuable mAbs along with other impurities.
Filtration
Filtration involves passing the bioreactor or culture medium through a filter to separate the target product from unwanted particles and impurities. Depending on the size and nature of the product, different types of filters, such as depth filters, membrane filters and chromatography columns, may be used.
Flocculation
Flocculation techniques utilize chemicals to aggregate cells or particles, making them easier to separate from the liquid medium. Flocculants cause the target product and impurities to form larger clumps or flocs, which can be separated by methods like centrifugation or filtration.
Foam Fractionation
Foam fractionation relies on the fact that certain components have a tendency to concentrate in the foam. The process involves generating a foam that rises and captures the desired product, while leaving impurities behind. The collected foam is then processed to recover the target product.
Precipitation
Precipitation involves adding salts, organic solvents or other substances to the culture or fermentation broth. This causes the desired product to form insoluble complexes that can be separated by centrifugation or filtration. Additional purification steps may be necessary to eliminate impurities linked to the precipitate.
Factors To Consider When Choosing a Method
When selecting a harvesting technique for downstream processing in biopharmaceutical manufacturing, several important factors should be considered. These factors include the nature of the product, cell or biomass type, scale of production, downstream processes, contamination removal and regulatory compliance. Biopharmaceutical manufacturers can choose a suitable harvesting technique that ensures efficient separation, high product yield and compliance with downstream processing steps and regulatory standards.
Nature of the Product
When deciding on a harvesting technique, it’s important to consider the specific characteristics of the product you’re trying to obtain. Factors such as stability, solubility, sensitivity to mechanical stress and vulnerability to degradation or denaturation are key in determining the appropriate method to use.
For products that are sensitive to shear forces, like delicate proteins or fragile biomolecules, it’s preferable to use gentle harvesting techniques such as filtration or sedimentation. These methods minimize the application of mechanical stress and reduce the risk of damaging or altering the product.
By selecting techniques that align with the specific characteristics of the product, biopharmaceutical manufacturers can ensure that the integrity of the product is maintained and the desired quality is preserved throughout the subsequent processing steps.
Cell or Biomass Type
Various types of cells, such as bacteria, yeast and mammalian cells, have different characteristics that require specific methods for harvesting. Bacterial cells have a strong cell wall made of a substance called peptidoglycan, which provides them with structural support. Yeast cells, which are also microorganisms, have a cell wall similar to bacteria but made of different substances, like glucans and mannoproteins.
Mammalian cells, which are widely used in biopharmaceutical production, have a more complex structure compared to bacteria and yeast. Depending on the specific type of cell or biomass being harvested, effective separation may require methods like enzymatic lysis (breaking down the cells using enzymes) or mechanical disruption techniques.
Scale of Production
The scale of the production operation is an important factor when deciding how to harvest materials. In small-scale manufacturing or laboratory settings, simple methods like using a centrifuge or filtration can be used, as they require readily available equipment. These methods efficiently separate materials and can be done on a smaller scale.
However, when it comes to large-scale manufacturing, different methods are needed that can handle larger quantities of materials. In industrial settings, techniques like continuous centrifugation, cross-flow filtration or large-scale flocculation systems are commonly used. These methods are designed to be scalable and cost-effective for handling large volumes of materials.
Downstream Processes
When choosing a harvesting technique, it’s important to consider how well it will work with subsequent purification, separation or formulation steps. The compatibility between the harvesting technique and downstream processes like chromatography or filtration can have a significant impact on the overall efficiency and yield of the entire process.
For instance, if chromatography is planned as a subsequent purification step, it’s beneficial to select a harvesting technique that produces a clear and relatively pure product stream. This helps streamline the purification process and improves efficiency. Similarly, if filtration is going to be used for further separation or concentration, it’s beneficial to choose a harvesting technique that produces a clear liquid phase. This enhances the effectiveness and productivity of the filtration step.
Contaminant Removal
Effectively removing impurities from the harvested material is important when going ahead with a harvesting technique. Impurities like host cell proteins, DNA or components from the culture media can negatively impact the purity and quality of the final product. The selected technique should have the capability to minimize the presence of these impurities in the harvested material.
For instance, centrifugation methods can separate solid particles such as cells and debris from the liquid phase, thereby reducing the concentration of host cell proteins and DNA. Filtration techniques with appropriate pore sizes can effectively remove particulate matter and larger impurities. Additionally, techniques like flocculation or precipitation can help aggregate and remove specific impurities.
Regulatory Compliance
Aligning with regulatory requirements regarding product safety, purity and quality is important when opting for a harvesting technique. The selected technique should comply with relevant regulations and guidelines to ensure that the harvested material meets the required standards.
It’s important to consider documentation and validation requirements, as well as the ease of implementing the technique in a regulated environment. Robust documentation, including standard operating procedures (SOPs) and batch records, should be in place to demonstrate adherence to regulatory standards. Validation studies may be necessary to confirm the effectiveness and consistency of the chosen technique in removing impurities and maintaining product quality.
Furthermore, the ease of implementation, including training requirements and the availability of qualified personnel, should be evaluated to ensure a smooth integration of the harvesting technique into the overall manufacturing process.
Cell lysis and clarification
Cell lysis and clarification are critical steps in downstream processing, particularly in the extraction of intracellular components like proteins, enzymes or nucleic acids from cells. The process involves breaking open the cell membrane to release the desired intracellular contents, while clarification methods are employed to remove cell debris and impurities from the resulting lysate. There are different techniques used for cell lysis, including mechanical and chemical methods:
Mechanical Cell Lysis: Mechanical methods physically disrupt the cell membrane to release intracellular components. Common mechanical techniques include homogenization, bead milling, sonication and high-pressure homogenization. These methods apply shear forces, pressure or cavitation to break the cell wall or membrane.
Chemical Cell Lysis: Chemical methods use chemicals to disrupt the cell membrane and release the intracellular contents. Examples include detergents like Triton X-100 or sodium dodecyl sulfate (SDS), which solubilize the cell membrane and facilitate the release of intracellular components. Enzymes such as lysozyme can also be used to degrade the cell wall in bacteria.
These techniques play a crucial role in isolating and extracting intracellular components for various applications in research, biotechnology and pharmaceutical production.
Challenges During Cell Lysis and Clarification
Cell lysis and clarification processes come with their own set of challenges that need to be addressed for successful outcomes. These challenges can impact the integrity of the sample, the selectivity and specificity of the process, scalability, cost-effectiveness and compatibility with downstream processes.
Maintaining Sample Integrity
Maintaining the integrity of the sample is crucial to preserve the target molecules during cell lysis and clarification processes. If sample integrity is not ensured during cell lysis and clarification processes, several undesirable consequences can occur, including degradation of target molecules, denaturation of proteins, contamination and loss of nucleic acid integrity.
Selectivity and Specificity
Selectivity and specificity are crucial factors to consider in cell lysis and clarification processes, especially when targeting specific cell types or components. Depending on the application, it may be necessary to selectively lyse certain cell populations while leaving others intact, or to separate specific components from the lysate.
If selectivity and specificity are not ensured during cell lysis and clarification processes, it can lead to several undesirable outcomes and limitations, including contamination, loss of target molecules, reduced purity and unnecessary interference with downstream processes.
Scalability
Scalability is a major challenge in cell lysis and clarification processes, as the techniques employed must be adaptable to handle different sample volumes and meet varying throughput requirements. Methods used in small-scale research experiments may not directly translate to large-scale industrial processes.
If scalability is not ensured in lysis and clarification techniques, it can lead to various limitations, including inefficient processing, inconsistent results, high costs and workflow integration issues. Therefore, ensuring consistent and efficient cell lysis and clarification across different scales is crucial to maintain the integrity and quality of the target molecules.
Cost-Effectiveness
Cost-effectiveness is an important consideration when selecting cell lysis and clarification methods, particularly in large-scale applications. If cost-effectiveness is not ensured when selecting cell lysis and clarification methods, several potential consequences can arise, including limited scalability, inefficient resource utilization, compromised quality and longer processing times. Therefore, it is important to strike a balance between the cost of the method and the desired level of clarification to optimize both financial and operational outcomes.
Compatibility With Downstream Processes
Compatibility with downstream processes is crucial to ensure efficient integration of the overall workflow and maximize the yield and quality of the target molecules. The chosen lysis and clarification methods should not interfere with subsequent steps, such as chromatography, filtration or downstream analysis techniques. If compatibility is not taken into account, several issues can arise, including loss of target molecules, interference with purification steps, damage to chromatography resins and inaccurate downstream analysis.
Types of chromatography used in downstream processing
Chromatography is a widely used technique in downstream bioprocessing that allows for the separation, purification and isolation of biomolecules. Different types of chromatography methods are employed to target specific components based on their physicochemical properties. Here are some common types of chromatography used in downstream processing:
Affinity Chromatography
This method utilizes the specific binding between a target biomolecule and an immobilized ligand. The stationary phase has a ligand that captures the target molecule selectively, resulting in high selectivity and purification efficiency. It is commonly used for isolating proteins, enzymes, antibodies and other biomolecules.
Ion Exchange Chromatography
This technique separates biomolecules based on their charge properties. The stationary phase contains resins with charged functional groups. Biomolecules with opposite charges to the resin selectively bind, and elution is achieved by adjusting the pH or ionic strength of the mobile phase. It is effective for purifying proteins, nucleic acids and other charged biomolecules.
Size Exclusion Chromatography
SEC separates biomolecules based on their size or molecular weight. The stationary phase consists of porous beads with different pore sizes. Larger molecules elute faster, as they cannot enter the pores, while smaller molecules penetrate the pores and elute later. It is useful for desalting, fractionation and purification of proteins, polysaccharides and nucleic acids.
Hydrophobic Interaction Chromatography
HIC separates biomolecules based on their hydrophobicity. The stationary phase contains a hydrophobic ligand, and binding occurs under high-salt conditions. As the salt concentration decreases, hydrophobic target molecules are eluted in order of decreasing hydrophobicity. HIC is commonly used for protein purification to remove aggregates and host cell proteins.
Reverse Phase Chromatography
RPC is used to purify hydrophobic molecules. The stationary phase is nonpolar, while the mobile phase is polar. Hydrophobic molecules interact with the stationary phase and are retained, while polar molecules elute earlier. RPC is commonly employed for purifying lipids, peptides, small proteins and hydrophobic compounds.
Steps in the Chromatography Process
The chromatography process involves several important steps to separate and purify biomolecules. These steps provide a general overview of the chromatography process. However, it’s important to note that the specific details and variations in each step can vary depending on the chromatography technique used, the nature of the biomolecules being studied and the purification objectives.
- Equilibration
The first step is to prepare the stationary phase of the chromatography column. This is done by treating it with a buffer or solvent system that matches the separation requirements. The purpose of this step is to ensure that the stationary phase is in the best condition to bind and release the biomolecules effectively.
- Sample Application
The sample containing the biomolecules of interest is carefully applied to the chromatography column. This can be done using techniques like injection, loading or pipetting, depending on the specific system. The sample is typically introduced at the top of the column, allowing it to interact with the stationary phase.
- Binding
In this step, the biomolecules in the sample interact with the stationary phase. The binding occurs based on the specific properties of the stationary phase and the target molecules. For example, in affinity chromatography, the target biomolecules selectively bind to an immobilized ligand, while in ion exchange chromatography, the binding is driven by charge interactions.
- Washing
Once the sample has been applied and the target molecules have bound to the stationary phase, any unbound or nonspecifically bound molecules are removed by washing the column with a suitable buffer or solvent system. This step helps eliminate impurities and contaminants while retaining the desired molecules.
- Elution
Elution is the process of selectively releasing the bound target molecules from the stationary phase. It involves using elution buffers or solvents that disrupt the interactions between the target molecules and the stationary phase. The choice of elution conditions depends on the specific type of chromatography and the purification goals. Elution can be achieved by changing factors like pH, ionic strength, or polarity of the mobile phase.
- Collection
As the elution buffer passes through the column, the target biomolecules are released and collected in separate fractions. These fractions contain purified target molecules that can be further analyzed, characterized or used for other purposes.
- Regeneration
After elution, the column is regenerated to restore its original state and remove any remaining bound molecules or contaminants. Regeneration typically involves washing the column with appropriate cleaning solutions or performing additional equilibration steps to prepare it for subsequent runs.
Strategies for Optimizing Yield and Purity
Optimizing yield and purity is important in chromatography to obtain high-quality purified biomolecules. That’s why researchers must employ some optimization strategies to enhance yield and purity in chromatography, resulting in high-quality purified biomolecules for downstream applications such as biochemical assays, structural studies or therapeutic development.
Sample Preparation
Proper handling and preparation of the starting material are essential to avoid degradation or contamination. Filtration or centrifugation can be used to remove debris or particulates that may interfere with the chromatography process. Maintaining appropriate storage conditions, such as temperature and pH, helps preserve sample integrity and stability, ensuring optimal yield and purity of the target biomolecules.
Optimized Binding Conditions
It is important to optimize the binding conditions, including pH, ionic strength and temperature, to enhance the interaction between the target molecules and the stationary phase. Finding the optimal conditions for specific interactions, such as affinity or ion exchange, improves binding affinity and selectivity, resulting in higher yield and purity of the desired molecules.
Washing and Elution Optimization
The washing step is critical for removing impurities while retaining the target molecules. Optimization of washing conditions, such as buffer composition, flow rate and volume, minimizes nonspecific binding and maximizes purity. Similarly, optimizing elution conditions ensures the selective release of the target molecules without significant degradation or loss, further enhancing yield and purity.
Column Selection and Parameters
Careful selection of the appropriate column, considering factors such as particle size, pore size and column dimensions, is crucial for achieving optimal yield and purity. The right column choice enables proper resolution and separation of target molecules, minimizing sample loss or contamination during the chromatography process.
Gradient Optimization
Gradient optimization is particularly important in techniques like gradient elution. Fine-tuning the gradient slope, duration and composition allows for efficient elution of the target molecules while maintaining stability and minimizing nonspecific binding. Proper gradient optimization enhances yield and purity by effectively separating the target molecules from unwanted impurities.
Process Monitoring and Control
Real-time monitoring of the chromatography process using techniques like UV spectroscopy, conductivity measurements or affinity assays enables immediate assessment of yield and purity. Continuous monitoring allows for on-the-fly adjustments to optimize purification parameters, ensuring maximum yield and purity while minimizing losses or deviations.
Recovery and Concentration
After elution, employing appropriate recovery and concentration techniques such as ultrafiltration, precipitation or dialysis facilitates the concentration of the purified biomolecules while eliminating residual contaminants or buffer components. Effective recovery and concentration steps enhance the overall yield and purity of the target molecules.
Column Regeneration and Maintenance
Proper column regeneration and maintenance protocols are vital to maintain column performance over multiple runs. Thorough cleaning and regeneration steps remove residual impurities or molecules, ensuring consistent yield and purity in subsequent chromatography trials. Additionally, adequate column maintenance contributes to the longevity and efficiency of the chromatography process.
Ultrafiltration and Diafiltration
Ultrafiltration and diafiltration are two important processes used in various industries for filtration and separation purposes. They are commonly employed in biopharmaceutical manufacturing, food and beverage processing and wastewater treatment.
Ultrafiltration is a filtration technique that uses a special membrane to separate particles and solutes based on their size. The membrane has tiny pores ranging from 1 to 100 nanometers. It allows small molecules like water, salts and low molecular weight solutes to pass through, while larger molecules such as proteins, colloids and suspended particles are retained. This separation is achieved by applying pressure to drive the liquid and small solutes through the membrane, while the larger molecules are trapped.
Diafiltration, also known as buffer exchange, is a process that combines ultrafiltration with the addition of a diafiltration buffer to effectively remove small molecules and contaminants from a solution. In diafiltration, the solution is first concentrated using ultrafiltration to reduce its volume and increase the concentration of the desired molecules. Then, a diafiltration buffer is added to the concentrated solution, and the process continues to replace the smaller molecules and contaminants with the buffer. This buffer exchange is achieved by continuously adding fresh buffer and removing the permeate from the system, gradually reducing the concentration of small molecules and contaminants.
Factors That Can Influence the Efficiency of These Processes
Several factors can influence the efficiency of ultrafiltration and diafiltration processes. By considering these factors and following the concerned optimization tips, the efficiency of processes involving membrane separation can be significantly enhanced, leading to improved productivity, cost-effectiveness and overall performance.
Membrane Selection
Choosing the right membrane material is important, as it determines how well the separation process will work. Factors to consider include permeability, selectivity, how strong the membrane is, its resistance to fouling and whether it is compatible with the fluids used in the process. By carefully evaluating different membrane options and selecting the most suitable one for the specific process, we can greatly improve efficiency.
Operating Conditions
Optimizing the operating conditions of the filtration process is essential for maximizing efficiency. Factors such as pressure, temperature, flow rates and concentration gradients can affect how well substances are separated. By adjusting these parameters according to the specific process requirements, we can achieve better separation performance. It’s important to find a balance between maximizing the flow rate of substances through the membrane and minimizing energy consumption or fouling.
Feed Pretreatment
Preparing the feed stream before filtration can enhance process efficiency by reducing the likelihood of fouling and scaling. This involves performing filtration or other separation steps to remove particles, suspended solids or contaminants from the feed. Effective pretreatment strategies can minimize membrane fouling, which leads to higher productivity and extends the life span of the membrane.
Membrane Fouling Control
Membrane fouling occurs when unwanted materials accumulate on the surface of the membrane, reducing its efficiency over time. To combat fouling, it’s important to implement appropriate control measures, such as regular cleaning, backwashing (reversing the flow) or using coatings that prevent fouling. These measures help mitigate the negative effects of fouling and maintain the efficiency of the filtration process.
System Monitoring and Control
Continuous monitoring of key process parameters is essential for optimizing efficiency. Monitoring variables like pressure differences, flow rates and the quality of the filtered substance can provide valuable insights into the performance of the system. By using automation and feedback control systems, we can make real-time adjustments to maintain optimal operating conditions, thereby maximizing efficiency.
Scale-Up Considerations
When scaling up a filtration process, it’s important to consider factors like the size of the membrane, the dynamics of flow and the design of the system. Properly designed and engineered systems can prevent issues such as concentration polarization (uneven distribution of substances), uneven flow distribution or excessive pressure drop. Taking these factors into account ensures that the filtration process operates efficiently when performed on a larger scale.
Formulation and Storage
In the biopharmaceutical industry, formulation plays a crucial role in preparing the final product with the desired composition, stability and dosage form. The process involves carefully selecting the right excipients, optimizing drug concentrations and determining the most suitable delivery system. These efforts are made to enhance the properties of the product, extend its shelf life and ensure its efficacy and safety during storage and administration.
Additionally, it is vital to store biopharmaceutical products under proper conditions, such as by controlling temperature and protecting them from light and moisture. These measures are necessary to maintain the stability and quality of the products. The formulation process and stringent storage conditions work together to maximize the therapeutic value and ensure patient safety when using biopharmaceuticals.
Formulation Techniques:
Buffering and pH Adjustment
Buffering agents are used in biopharmaceutical formulations to maintain a specific pH range. PH plays a crucial role in the stability and activity of biopharmaceuticals. Buffering agents help resist changes in pH by absorbing excess acids or bases, ensuring the desired pH conditions are maintained. This is important because biopharmaceuticals can be sensitive to pH variations, which can affect their structure, solubility and efficacy. By carefully selecting suitable buffering agents and adjusting pH, the formulation provides optimal conditions for the biopharmaceutical to remain stable and retain its activity during storage and administration.
Stabilizers and Excipients
Stabilizers and excipients are key components of biopharmaceutical formulations, as they enhance stability, prevent degradation and maintain the activity of the active ingredient. Stabilizers can include sugars, polyols, surfactants, preservatives and antioxidants. These additives protect the biopharmaceutical from degradation processes like oxidation, hydrolysis and denaturation that can occur during manufacturing, storage or administration. Excipients, on the other hand, are inert substances added to formulations to improve drug delivery, solubility and other formulation properties. They affect factors like viscosity, osmolarity and tonicity, ensuring compatibility with the intended route of administration and patient acceptance.
Cryoprotectants
Cryoprotectants are vital for long-term storage of biopharmaceutical products, especially those that undergo freezing or lyophilization (freeze-drying). These agents protect the product from damage caused by ice crystal formation during freezing and dehydration during drying. Cryoprotectants lower the freezing point, preventing the formation of large ice crystals that could harm the biopharmaceutical’s structure. They also provide stability during drying by maintaining the product’s physical and chemical integrity. Common cryoprotectants include sugars, polyols, amino acids and certain proteins, which effectively safeguard biopharmaceuticals during freezing and drying, enabling long-term storage and reconstitution when needed.
Formulation Optimization
Formulation optimization is a critical step in biopharmaceutical development, involving the evaluation and adjustment of various parameters to achieve desired product stability, solubility and efficacy. This process considers factors such as pH, temperature, concentration and excipient composition. By systematically varying these parameters, formulation scientists can assess their impact on the biopharmaceutical’s properties and activity. Through careful optimization, an ideal formulation can be developed that provides the required stability, release profile and physical characteristics suitable for the intended route of administration. Formulation optimization ensures that the biopharmaceutical product maintains its integrity and therapeutic effectiveness throughout its shelf life and patient use.
Storage Options
Refrigeration
Many biopharmaceutical products, such as vaccines and some biologics, are stored under refrigerated conditions (2-8°C). Cold storage slows down degradation processes, such as enzyme activity and chemical reactions, helping maintain the integrity and stability of the product for shorter periods. It is crucial to ensure that refrigerated products are stored within the recommended temperature range to avoid compromising their efficacy and safety.
Freezing
Certain biopharmaceuticals, including some cell therapies and plasma-derived products, require long-term storage at ultralow temperatures, typically below -20°C or even below -80°C. Freezing helps preserve the stability of these products and extends their shelf life by significantly reducing degradation processes. Proper packaging and storage equipment, such as cryogenic freezers, are necessary to maintain the required low temperatures and prevent damage to the product.
Lyophilization
Lyophilization, or freeze-drying, is a process commonly used for biopharmaceuticals that are unstable in liquid form. It involves removing water from the product by sublimation under vacuum. Lyophilized products have extended stability, allowing storage at ambient temperatures, and can be reconstituted prior to use by adding a suitable solvent. This technique is particularly beneficial for products that need to be transported, stored or reconstituted easily while maintaining their efficacy and stability.
Controlled Room Temperature
Some stable biopharmaceutical products can be stored at controlled room temperature (CRT), typically between 15-25°C, for a defined period. The storage conditions at CRT are determined through stability studies and regulatory guidelines, ensuring that the product maintains its quality and effectiveness within the specified time frame. It is important to monitor environmental factors, such as humidity and light exposure, to prevent degradation and ensure product stability during storage at room temperature.
Best practices for optimizing downstream processing techniques
Optimizing downstream processing techniques is crucial for efficient and cost-effective production of biopharmaceuticals. By following best practices, biopharmaceutical companies can enhance the yield, purity and overall quality of their products.
Robust Process Development
Thorough process development is crucial to establish a robust downstream processing strategy. This involves a deep understanding of the target molecule’s characteristics, such as solubility, stability and interactions, which guides the selection of appropriate techniques. Optimizing parameters like pH, temperature and flow rates ensures efficient and consistent purification. Robust process development minimizes variability and enhances scalability.
Use of High-Quality Starting Materials
Starting with high-quality materials, including well-characterized cell lines, culture media and raw materials, is essential. Quality materials lead to higher yields and reduce impurities that could affect product quality and efficacy. Implementing robust supplier qualification and material characterization processes ensures consistency and traceability of starting materials.
Quality by Design
Quality by Design (QbD) is a systematic approach that aims to ensure the desired quality of the final product by designing and controlling the manufacturing process. It involves identifying critical quality attributes (CQAs) of the product and linking them to critical process parameters (CPPs) to establish a design space. QbD utilizes risk assessment tools, such as failure mode and effects analysis (FMEA) and design of experiments (DOE), to mitigate potential risks and optimize process performance. Implementing QbD in downstream processing offers benefits such as enhanced process understanding, increased process robustness and improved product quality and consistency.
Continuous Monitoring and Control
Real-time monitoring and control of critical process parameters enable immediate response to deviations and ensure consistency and reproducibility. Monitoring pH, temperature, pressure and flow rates during purification steps allows for timely adjustments and optimization, minimizing variability and ensuring product quality.
Integration of Process Steps
Process integration and automation minimize manual interventions, reduce handling steps and improve efficiency. By seamlessly connecting different process steps, the risk of contamination or product loss is minimized and productivity is increased. Integration streamlines the workflow, reduces human error and accelerates time to market.
Future considerations for optimizing downstream processes
With continuous technological advancements, there are several future considerations to optimize downstream processing techniques in the biopharmaceutical industry. These considerations include the development of novel separation and purification techniques, membrane-based separations and continuous processing systems.
Process Analytical Technology
Process analytical technology (PAT) is an invaluable tool for optimizing downstream processing techniques. By implementing advanced PAT tools, such as real-time monitoring sensors and data analysis techniques, manufacturers can gain valuable insights into process variability. This enables them to intervene and optimize the process in a timely manner, ensuring consistent product quality. PAT facilitates data-driven decision-making, improves process understanding and enhances control over critical process parameters and quality attributes.
Single-Use Technologies
Single-use technologies offer numerous benefits in downstream processing. By utilizing disposable chromatography columns, filtration systems and other single-use equipment, manufacturers can reduce the risk of cross-contamination and eliminate the need for extensive cleaning and validation processes. Additionally, single-use technologies provide flexibility in manufacturing operations, allowing for easier process scale-up, faster turnaround times and simplified facility design. They also contribute to reducing water and energy consumption, making the manufacturing process more sustainable.
Continuous Processing
Continuous Processing has gained significant attention in recent years. By adopting continuous downstream bioprocessing, manufacturers can achieve higher productivity and efficiency. Continuous systems enable a continuous flow of materials, eliminating batch-to-batch variations and reducing processing time. This approach offers benefits such as higher throughput, improved process control and reduced footprint. Continuous processing also supports the implementation of real-time monitoring and control strategies, leading to enhanced process robustness and consistent product quality.
Learn more about downstream process optimization with Avantor’s expert support! Discover cutting-edge solutions and unlock efficiency gains. You can refer to this resource to access Avantor’s comprehensive solutions and take your operations to the next level.